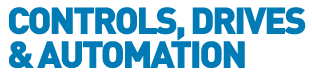
![]() |
Charlotte Stonestreet
Managing Editor |
How to make a robot application safe
21 October 2019
In the first CDA webinar, David Collier, Certified Machinery Safety Expert from Pilz looked at how to to make a robot application safe, with particular emphasis on collaborative robots
When industrial robots first started to become commonplace in the early 1970s, the earliest multi-access units tended to have no incorporated safety technology. Today, the basic safety concepts applied to modern day robots either enclose units behind safe guarding with interlocked access points or utilise a cell where, rather than the robot being enclosed, there is an opening which is guarded using technology such as light curtains, laser scanners, or even radar.
However, over the last few years the development of collaborative robots, or cobots, has changed the way we look at human/robot interaction in the industrial environment.
“A cobot is basically a robot for collaborative operations where the robot will occupy the same space as the human being; where there is a potential for a collision between the moving robot and the person,” says David Collier, Certified Machine Safety Expert at Pilz. “So this implies we are no longer looking at physical guarding or detection systems which are external to the robot, we are now looking at robots which have built-in sensing capabilities, the simplest of which would be springs and clutches, the more complex of which would include torque sensors or monitoring currents in each of the individual motors in each axis of the robot.
“A robot has at least one intelligent safety function. Most industrial robots will have the ability to be emergency stopped through external signals, but a cobot will also have intelligent safety functions built in such as force limitation. So that implies the cobot is able to detect a collision, through which ever sensing technology is built in, and is able to detect when it makes impact with the human.”
In terms of the design and appearance of conventional industrial robots versus cobots, it is usually fairly simple to tell one from the other. Industrial robots tend to be larger, with greater payloads and have numerous potential trapping points. In contrast, cobots are typically ergonomically designed not to have any shop edges and any contact points that the robot might have with a human have quite large surface areas to limit the amount of pressure felt. Many cobot manufacturers have taken care whilst designing their units to minimise sheering and trapping points between axes.
When it comes to machinery safety, although a robot does not constitute a complete machine in its own right, once it is put into an application it is then classed as a ‘machine’ and needs to meet the requirements of the Machinery Directive, conformity to which is through the use of standards known as normative requirements.
One of most referenced documents when it comes to robotics, and in particular cobotics, EN ISO 10218-2 is the standard that is used by those who are integrating robots into an application. It is a Type C standard under the machinery directive, meaning it is a machine specific standard and it has quite a large range for various applications for types of robotics use. EN ISO 10218-2 includes Annex G which details over 200 safety points that need to be checked or validated to make sure that the application you are using the robot in is safe.
EN ISO 10218-2 also refers to ISO TS 15066 - Robots and robotic devices. This is a Technical Specification rather than a standard, and is designed to assist designers of robotics cells in making them safe. It offers very specific guidance on human robot collaboration, describing four methods, as well as a ‘body model’ which details 29 different points of the human body that might be impacted by a robot along with specific bio-mechanical limit values for each.
Human robot collaboration
Both EN ISO 10218-2 and ISO TS 15066 refer to four methods of human robot collaboration, the first of which is known as safety-rated stop. This is where there is, for instance, some kind of physical barrier with an interlock that when opened brings a robot to a stop, or a scanner is used to detect somebody approaching the robot, which then stops. In order to get into the collaborative workspace the robot must have been safely stopped, excluding the possibility of anybody coming into contact with the moving robot.
The second method, hand guiding, is often used in conjunction with safety-rated stop. Here, if a unit operating under safety-rated stop needs to be operated in teach mode, once it has been brought to standstill, it is possible to hand guide the robot while pressing an enabling switch.
“These enabling switches are very common on the market, you tend to find them as a standalone device or even on the back of the teach pendant for the robot,” says Collier. “The idea is that the operator can take the tool centre point of the robot to where it needs to be and even teach the robot to follow a certain path manually while they are holding the enabling switch. If anything goes wrong, they either squeeze the switch extra hard or let go of it, which will put it back into a safe stop mode.”
The third method is referred to as speed and separation monitoring. Similar to the safety-rated stop method, which could be using for example a laser scanner or even radar technology, there is open space around a robot. As a person approaches the moving robot, the sensing system detects them and automatically reduces the speed of the robot, so they are now entering into warning area, or the yellow zone; any closer and the robot will automatically stop.
Key to all three of these methods is that they do not permit collision between the moving robot and the human being. This is where method four – which applies particularly to cobots – power and force limiting, differs; physical contact between human and robot is possible, whether intentional or unintentional. And risk reduction isn’t achieved by bringing the robot to a standstill before you get close to it; it is done through a number of methods.
“As with all risk reduction strategies you should start with inherent safe design,” says Collier. “The cobot itself has inherent safe design built in; it has smooth edges, large contact surface areas. In the application, the end effector for example, you would not want a blade or a hot device or a laser because no matter how slowly it is moving and how sensitive to force and pressure it is, the end effector itself might just simply be too dangerous. This is where inherent safe design might need be applied by the integrator, not the robot manufacturer, for example, by applying shoring around the end effector.”
Further risk reduction comes from the safety functions which are built into the robot.
When it comes to the CE mark, which indicates that applicable EU requirements have been met, as a robot is not classed as a machine the CE mark found on a robot does not indicate compliance with the Machine Directive, and does not mean that it can be classified as safe. Even a cobot with force limiting cannot be classed as safe – indeed, no robot can be legally declared safe until the application has been risk assessed and all measures required to make it safe taken. Responsibility for this will generally lie with the integrator, or in the case of a complete machine, the manufacturer.
Robot safety lifecycle
Over many years Pilz has developed a lifecycle to making a robot safe. One of the most critical aspects of this is that before a robot or robot is purchased, the application in which it is to be integrated is fully understood. Fundamentally, is a collaborative space needed? Does the application really require a human to be in the same space as the robot when it’s moving, and if so what tasks will they need to perform? Does the robot ned to be active during these phases?
Other considerations are the type of material the robot will be handling, the end effector, and the tool piece, as well as cycle times and kinematics. It is also important to look at steps needed to avoid avoid singularity; the circumstances in which the robot might behave unexpectedly.
Understanding the application right from the very beginning may identify factors which preclude the use of a cobot entirely, for example a requirement for high cycle times may mean a robot moves too fast for collaborative use.
“Once you’ve understood the application, then you need to look at a risk assessment, the basic document for the CE marking process. It involves defining the limits of the machine in which the robot its going to be used in terms of space and time, for example, and how fast it’s going to be moving, what it’s going to be handling, what tools are going to be used on the end of it,” says Collier.
“You need to identify the different types of collision that might take place, so typically if it’s a cobotic application you need to look at whether someone is just going to be hit by the robot, or even clamped or trapped by it, these are known as either transient or quasi-static impacts. You need to consider not only what happens when somebody approaches the robot while it is moving, but also once it is stood still what might happen if it inadvertently starts up whilst someone is in the same workspace, which is known as unexpected start-up.”
Having identified all the potential hazards of the different phases of the robot’s use or misuse, the risk assessment is used as a basis upon which to draw up concepts on how to make the application safe.
“Risk reduction is normally carried out via a three stage method: first of all trying to design hazards out through inherent safe design, then through functional safety and safe guarding to reduce risks – not to zero but try to control them in a safe way – and finally by providing user information,” says Collier.
Inherent safe design
Inherent safe design involves making sure that not only the cobot but also the tool piece and any parts that it might be picking up have rounded edges and corners, or there may be padding. Inherent safe design can also involve making sure that the path the robot follows isn’t going to trap anybody. Depending on the tasks that are involved, gaps need to be big enough to allow parts of the body not to be crushed. Also, if the robot were to drop something, rather than allowing a person to bend over or crouch down and expose their head to a moving part of the robot, it is recommended to provide some kind of tool for retrieving lost pieces.
The functional safety aspect involves optimising the robot path to avoid parts of the body being crushed, or using the the safety rated features to reduce the dynamics of the robot, such as speed, force power and torque. With some cobots it is also possible to restrict the collaboration space itself, vertically and horizontally.
The last step in risk reduction – accepting the fact that there will always be some residual risk – is to provide the user with information. For example, you might want to mark out on the floor where the collaborative space is. The operating manual needs to provide clear guidance on how operators are to interact with the cobot and also instructions on how to retrieve lost work pieces. It may also possible be to put signage in and around the robot cell, warning people of the hazards.
Once the safety concepts have been implemented and the cell is ready for commissioning, with a cobotic application there may be requirement to make overrun measurements, the limit values of which need to be validated. To aid in this, Pilz offers a robotic measuring system, which uses sensors to measure the force of impact that will be experienced by a person on different parts of the body should they come into contact with the cobot.
To learn more about any of the issues covered in this article and more, you can listen to the webinar in full via bit.ly/31NScWB
- When were your Light Curtains last tested?
- Safeguarding frequent access danger zones
- Safety locking devices
- Pilz launches new PUWER Assessment service
- Slimline safety switches
- Machine safety webinar series
- En route to robot safety
- Safety and security go hand in hand
- Pilz appoints new BDM for motion control products
- Series of Machinery Safety Courses this June from Pilz UK
- No related articles listed